A bird’s exterior can be described by dividing it into main parts such as the head, neck, trunk, tail, wing (or thoracic limb), and pelvic limb.1 These parts can then be subdivided into regions to create ornithological descriptions and thus used to study birds in which some terms are widely employed while others have fallen into disuse.1 Morphologically, there is uniformity among birds due to flight constraints in which avian skeletons are modified for flying. Bird bones are lighter in weight than mammalian bones because some are hollow, termed pneumatic bones (e.g., skull, humerus, clavicle, keel, pelvic girdle, lumbar and sacral vertebrate). Additionally, birds have a lightweight fused skeleton.2 This article focuses on the basics of musculoskeletal anatomy in birds. Instead of an in-depth discussion of anatomy, some of the birds’ unique musculoskeletal features and functions are highlighted.
Axial skeleton
Skull
The avian skull consists of a honeycomb of air spaces with delicate spicules for support in which the size of the head has been reduced significantly compared to other species.2 The size of the head may represent a feature of the avian skeleton modified to accommodate the constraints of flight.
Cervical vertebrae
The avian neck is regarded as a classic example of high variability ranging from short necks in songbirds to extremely long, serpentine necks in herons.3 The soft tissues of the neck, the esophagus, and the trachea are shorter than the cervical vertebra. Because of this, it is impossible to stretch a bird’s neck out completely.2 To protect the delicate tissues of the brain, the flexibility of the neck acts as a shock absorber, in which, in most species, the neck is quite long. The neck length varies with the number of the cervical vertebra, which ranges from 11 to 25.1 The avian neck length helps the bird reach food located on the ground and adjust to the center of gravity when changing from an upright position of walking to a more horizontal position when flying. Notably, the minimal length of the neck must be long enough to enable the beak to reach the tail region with a uropygial gland. This preen gland provides oil used by birds to preen that is important for the maintenance of the feathers and addressing ectoparasites.1 In contrast with mammals, body size does not constrain neck length in birds. Instead, in birds, neck length scales isometrically with total leg length, suggesting a correlated evolution between both modules.3
Thoracic vertebrae
Thoracic vertebrae in birds vary from 3 to 10 and can be identified by ribs articulating with the sternum. Many bones of the avian backbone and limbs are fused to form a rigid and strong but light framework, including modified ribs. The uncinate process consists of extensions of bone projecting off the ribs that connect the ribs to the ones beside them. This fused rib cage then helps resist the twisting and bending of wings while flying and strengthens the rib cage so that it will not collapse during flight. It also adds strength to the thoracic wall acting as a protective brace around the chest and heart; and is particularly well developed in diving birds and thus helps the thorax withstand the pressure of a dive.2
Synsacrum
This fused series of vertebrae provides a rigid framework through which the limbs support the body. Although the exact boundaries between the vertebrae of these regions cannot be readily identified; the synsacrum consists of about 10-23 thoracic, lumbar, sacral, and caudal vertebrae.1
Caudal vertebrae
The final few caudal vertebrae of the avian skeleton are fused into a single ossification, the pygostyle.1 As such, this ossification consists of flattened bone to create a fused tail vertebra that forms a short tail for steering and maneuverability. The pygostyle is a crucial structure that supports the tail feathers and musculature most highly developed in birds that use their tails for climbing.2
Sternum
One of the largest bones in the modern avian skeleton is the sternum, a ventral plate of bone that provides protection.2 The sternum is keeled and thus extends outward and perpendicular to the plane of the ribs. As such, this keel bone is the living birds’ most important skeletal element and provides the main attachment for the flight muscles.4 It has many functions (almost all of which are thought to be related to flight) and is massive in flying birds and sophisticated flyers like swifts and hummingbirds.2, 4 It is reduced or absent in flightless birds such as emu, ostrich, and kiwi.
Appendicular skeleton
The pectoral girdle or thoracic girdle
The pectoral girdle in birds consists of rigid bones (e.g., the clavicle, coracoid, and scapula) that support the forelimb by acting as a wing strut that serves to brace the wings during flight.2
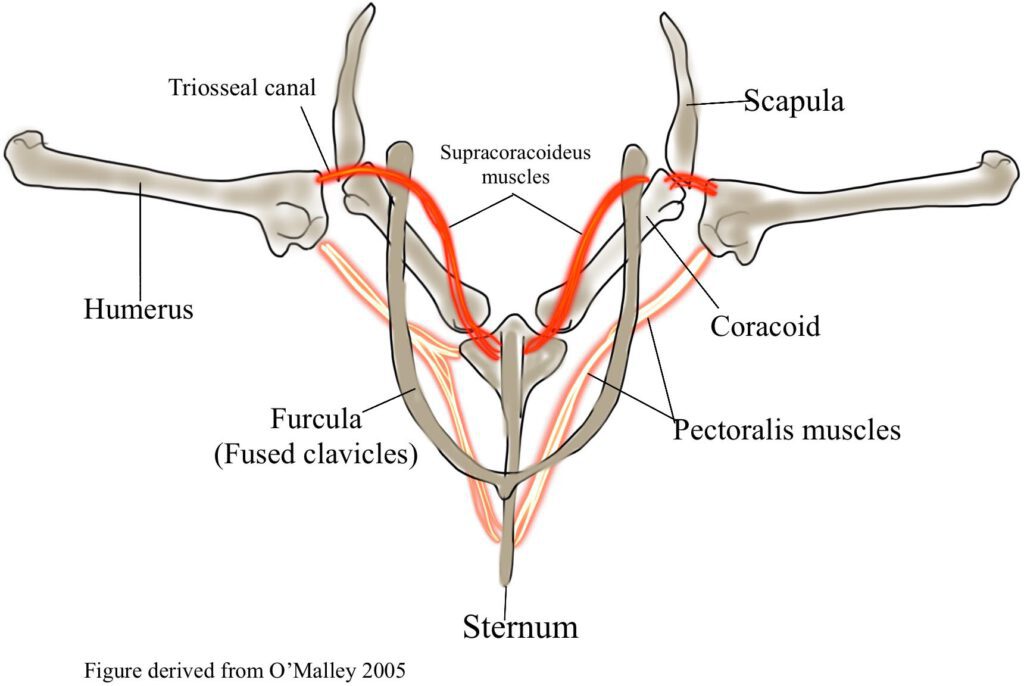
Clavicle
In the pectoral girdle, this bone supports the thoracic skeleton to help birds withstand the rigors of flight. Additionally, clavicles generally fuse ventrally to form the furcula or wishbone that provides extra strength.1 Birds are the only vertebrate animals with a fused collarbone that unites both shoulder blades resulting in a spring-like function.1 In many parrots and owls, the clavicles are united only by cartilage or fibrous tissue. However, the clavicles are reduced or absent in other parrots and ratites (e.g., ostrich, rhea, emu, cassowary, and kiwi). The clavicles remain separate and sometimes fused to the scapula or coracoid when present in ratites.1
Coracoid
This is a massive, short, strong bone in most birds and articulates firmly with the sternum acting as struts that hold the wings away from the sternum during flight.1 By doing this, the thoracic cage is protected from collapsing due to the powerful contractions of the pectoral muscles during the downstroke of the wings.1 In contrast, during gliding flight, the bones of the thoracic girdle, including the coracoids, act as a platform that bears the weight of the viscera. Importantly, the coracoid is commonly damaged during wing trauma. In this case, radiographs may reveal a coracoid bone fracture where the wing is drooping, and the humerus feels intact.
Scapula
A long bladelike bone that lies parallel to the backbone and extends as far caudal as the pelvis, with a length that varies with the flying strength of each avian species.2 As such, it is longest in strong flying birds, very small in flightless birds like the ostrich, and extremely broad in penguins which use their wings for swimming.1 Notably, the scapula and coracoid are fused in ratites.
Pelvic girdle
The pelvic limb includes a fusion of some bones and elimination of others, especially in the distal part of the limb.1 This means that there is variability in pelvic morphology across different orders of birds with various types of locomotion.5 Nevertheless, the pelvic girdle consists of the fusion of three paired elements, the ilium, ischium, and pubis.2 The pelvic girdle provides physical support and attachment for the hind limb musculature necessary for functions such as perching and locomotion like running and swimming.1, 2, 5
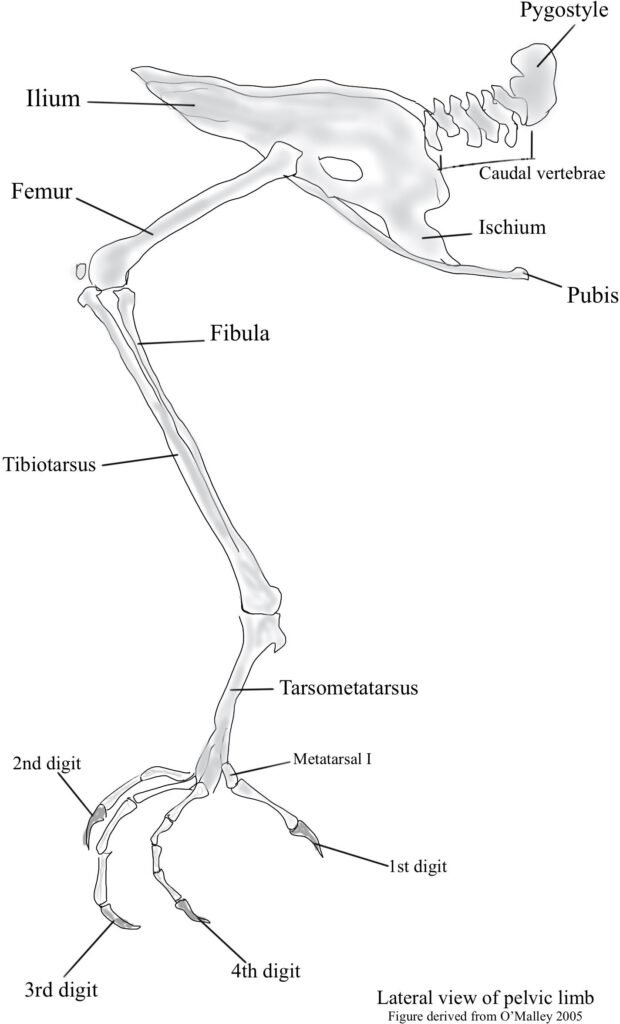
Wings
The wing skeleton characteristically consists of the humerus, radius, ulna, carpal bones, a carpometacarpus, and three digits.1
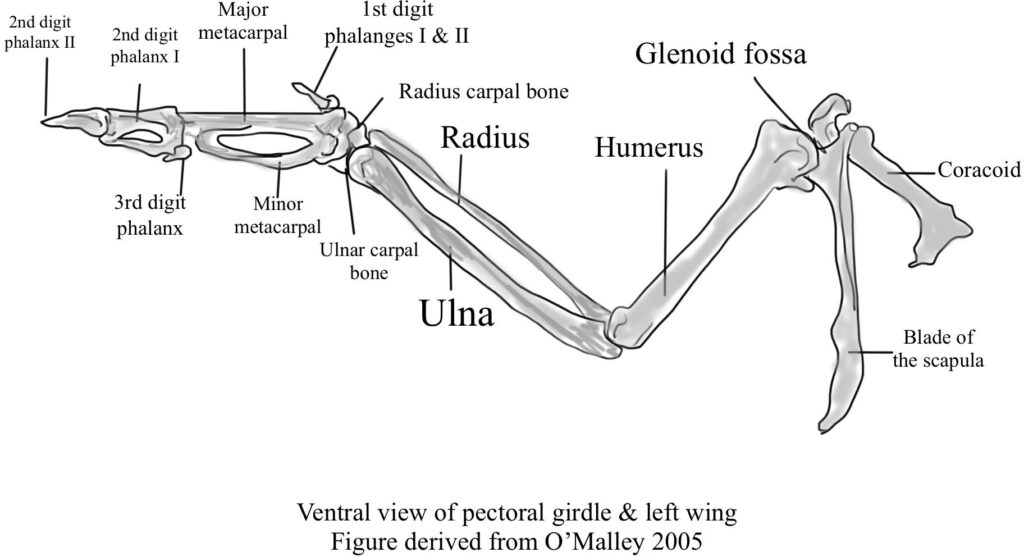
In addition, birds have four basic wing types: elliptical, broad, narrowed, and tapered.2
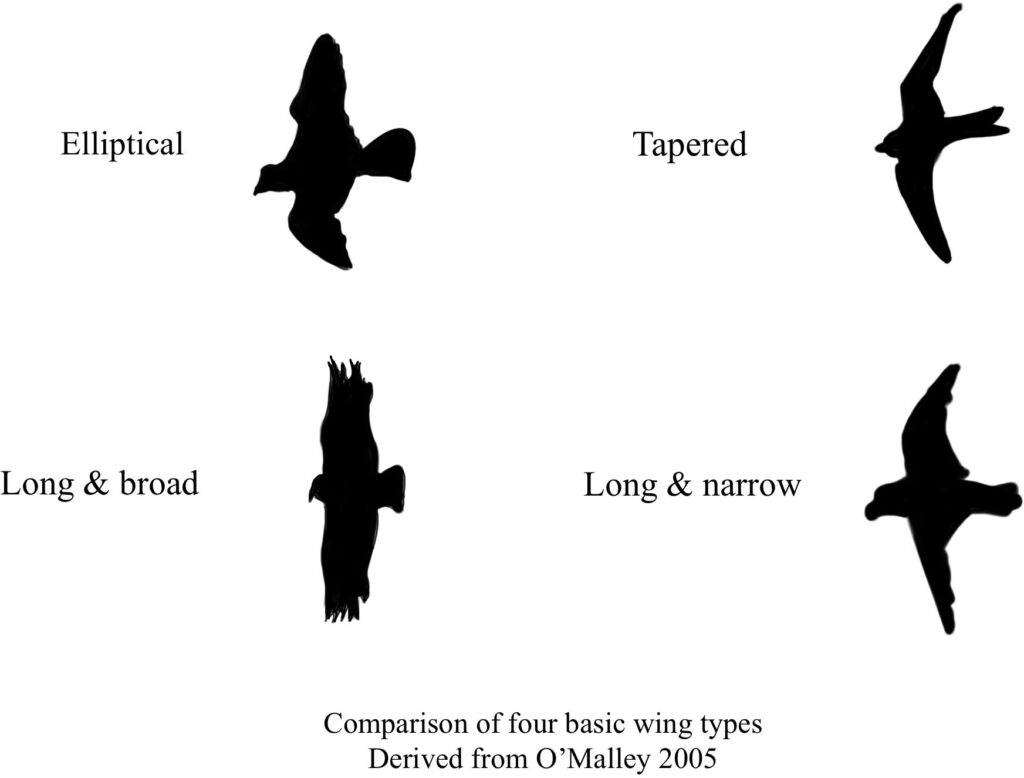
Importantly, when flight feathers spread out, they create “slots” between adjacent feathers. With these slots, birds catch hot air (i.e., thermals), allowing them to rise higher in the air. Passerines (e.g., robins, finches, chickadees, and sparrows) and pigeons (i.e., order Columbiformes) have short, broad elliptical wings with rapid beats to maneuver through the undergrowth.2 Eagles and vultures have long, broad wings and wing slots to soar at low speeds.2 Ocean-going birds like gulls and gannets have long, narrow, pointed wings without wing slots, allowing them to sore at high speeds. Swifts and falcons have tapered wings with no wing slots, allowing them to fly with rapid wing beads at high speeds.1, 2
Humerus
The humerus is a short bone that lies against the body when the wing is folded. It rotates away from the body during flight. Because of this motion, the dorsal edge of the wing at rest becomes the trailing edge during flight.1
Radius and ulna
These are long bones that lie parallel to each other in which the ulna is more massive than the radius.1, 2 The bones are widely separated, forming a slightly bowed unit along their length. Because of these features, the resistance to forces in the plane in which the bones lie increases. These bones are best developed in birds with wings specialized for soaring.1
Manus
The avian hand or manus is reduced through fused carpals and digits, and thus the avian forelimb is modified into a wing that holds the primary feathers.2 The manus in birds is thought to have evolved from the five-fingered hands and complex wrists of early dinosaurs representing one of the significant transformations of manus morphology among tetrapods.6
Musculature
Avian muscles consist of a mixture of white and red muscles, and the function of the muscle can be identified by its color.2 Myoglobin, an iron- and oxygen-binding protein found in vertebrates’ cardiac and skeletal muscle tissue, gives red muscles their color. Red muscles have an extensive blood supply and represent flight muscles that utilize aerobic metabolism, a chemical process in which oxygen is used to make energy from carbohydrates over a long period. In contrast, white muscles are powered by anaerobic metabolism, the process of creating energy without the presence of oxygen. White muscles are good for rapid contractions but tire quickly due to the build-up of lactic acid, the organic acid formed within muscle cells.
Muscles of flight
The massive keeled sternum provides an attachment surface for the powerful flight muscles (e.g., the m. supracoracoideus and m. pectoralis) (See figure 1). The supracoracoideus muscle contributes to lifting the wing during flight and is especially important during takeoff. In contrast, the pectoralis muscle is primarily responsible for the downstroke.4 In addition, the tail muscles, where the number can vary according to species, are also well developed for fine control of the tail feathers.2
Propatagium
It is a web of elastic fiber that stretches with wing extension to form the wing’s leading edge and a triangular fold of skin that extends from the shoulder to the carpus.7 The propatagium is essential for producing aerodynamic lift for flight.7
Pelvic limb
In general, the pelvic limb consists of the femur, tibiotarsus, fibula, tarsometatarsus, metatarsal bone, and in most birds, four digits.1 It is covered by feathers and skin as far as the level of the intertarsal joint and is followed by scales below.2 The pelvic limb generally is not involved in flight but is used more for swimming, catching prey, and wading.1, 2
Femur
In general, this is a stout short bone with a distal end that slopes cranially when the bird stands so that the limbs are brought forward on the body close to the bird’s center of gravity.1, 2
Tibiotarsus
It is the largest bone on the pelvic limb resulting from the fusion of the tibia and the proximal tarsal bones and is commonly called a drumstick in poultry.2
Tarsometatarsus
This bone is comprised of the fusion of the tarsal bones two, three, and four to the corresponding three main metatarsal bones (of digits II, III, and IV). The shape of the tarsometatarsus varies according to the locomotion of the species. For example, in most birds, it is shorter than the tibiotarsus. However, in long-legged birds like waders, the two bones are near equal in length.1, 2
Digits & Phalanges
Digits are formed by several bones called phalanges. The main action of the digits is flexion and extension and where most birds have four digits with varying numbers of phalanges.2 Birds utilize digitigrade locomotion because they walk or run on their toes and not on their feet. The arrangement of avian digits depends on the function and where feet can be used to perch, grasp prey, wade, or become fully webbed for swimming.2 Importantly, to reduce the energy needed for perching, the tendons of birds are flexed, and small sprocket-like projections extend from these tendons and interdigitate to hold them in place.2 A hallmark of the evolution of birds is the grasping capability of their feet. Nevertheless, the mechanics of avian foot function are not well understood.8 It has been suggested that two evolutionary trends contribute to the mechanical complexity of the avian foot. These trends are the variation in the relative lengths of the phalanges and the subdivision and variation of the digital flexor musculature observed among taxa.8
Anisodactyl
The first toe is directed backward, and the other three toes are directed forward, allowing the bird to adapt for perching or holding prey.2
Zygodactyl
The two toes point forward while the other two backward such as with owls and ospreys, with a basic zygodactyl foot but can move the fourth digit from back to front.2
Palmate
Cranial digits two, three, and four are webbed, such as the case with ducks, geese, swans, and gulls. Because of the palmate foot, the bird’s capacity to grip is sacrificed, and the backward-pointing digit loses contact with the ground.2
How do birds fly?
In adult birds, the flapping flight is the most power-demanding mode of locomotion, associated with a suite of anatomical specializations.8 In contrast, many developing birds use their forelimbs to negotiate environments long before acquiring “flight adaptations.”9 The idea is that developing birds recruit their developing wings to enhance leg performance continuously and, in some cases, fly.9 As previously mentioned, the supracoracoideus muscle contributes to lifting the wing during flight. It is especially important during takeoff, whereas the pectoralis muscle is primarily responsible for the downstroke.4 Accordingly, wings provide lift and propulsive force. Additionally, the primary wing feathers provide most propulsion, and the secondary feathers provide the lift.2 The ability to become rapidly airborne is seen in smaller birds. In contrast, large birds like the swan have to taxi along the water before they can take off.2 The bird’s dorsal surface of the wing is convex, and the ventral surface is concave.2 The leading edge is thickest, where bones and muscles provide strength and taper caudally to the trailing edge. Air traveling over the dorsal wing travels further than air passing ventrally. In this process, increased speed produces low pressure dorsally. As the air passes over the wing, it generates an upward force called lift, an aerodynamic force that enables the bird to rise into the air and defies gravity.2
References
- King, A. S., & Jonh Mclelland. (1984). Birds : their structure and function. Baillière Tindall.Millis, D. L., & Levine, D. (2014). Canine rehabilitation and physical therapy (2nd ed.). Saunders, Cop
- Bairbre O’malley. (2005). Clinical anatomy and physiology of exotic species : structure and function of mammals, birds, reptiles, and amphibians. Elsevier Saunders.
- Böhmer, C., Plateau, O., Cornette, R., & Abourachid, A. (2019). Correlated evolution of neck length and leg length in birds. Royal Society Open Science, 6(5), 181588. https://doi.org/10.1098/rsos.181588
- Zheng, X., Wang, X., O’Connor, J., & Zhou, Z. (2012). Insight into the early evolution of the avian sternum from juvenile enantiornithines. Nature Communications, 3(1). https://doi.org/10.1038/ncomms2104
- Anten-Houston, M. V., Ruta, M., & Deeming, D. C. (2017). Effects of phylogeny and locomotor style on the allometry of body mass and pelvic dimensions in birds. Journal of anatomy, 231(3), 342–358. https://doi.org/10.1111/joa.12647
- Barta, D. E., Nesbitt, S. J., & Norell, M. A. (2018). The evolution of the manus of early theropod dinosaurs is characterized by high inter- and intraspecific variation. Journal of Anatomy, 232(1), 80–104. https://doi.org/10.1111/joa.12719
- Samour, J. (2016). Avian medicine. Mosby.
- Backus, S. B., Sustaita, D., Odhner, L. U., & Dollar, A. M. (2015). Mechanical analysis of avian feet: multiarticular muscles in grasping and perching. Royal Society Open Science, 2(2), 140350. https://doi.org/10.1098/rsos.140350
- Heers, A. M., Rankin, J. W., & Hutchinson, J. R. (2018). Building a Bird: Musculoskeletal Modeling and Simulation of Wing-Assisted Incline Running During Avian Ontogeny. Frontiers in Bioengineering and Biotechnology, 6. https://doi.org/10.3389/fbioe.2018.00140